Research
What is strongly correlated-electron materials?
The Shimada-Ideta group at Hiroshima Synchrotron Radiation Center, Hiroshima University, focuses on researches using angle-resolved photoemission spectroscopy (ARPES) and related techniques to elucidate the electronic structure of strongly correlated-electron systems and so on. The electrons that we focus on exsit in materials and play an important role in their physical properties. For example, in metals that conduct electricity, electrons can move freely in the materials (free electrons), and this is what causes electricity to flow in the material. On the other hand, when the electron density becomes very high, they strongly interact with each other and their interactions with spin and orbital degrees of freedom make it impossible to explain electron motion using band theory, the basic theory of solid state physics. Because the electrons are strongly correlated with each other, such a system is called a "strongly correlated electron system". These materials show a variety of physical properties due to the complex interplay of electron degrees of freedom (charge, spin, and orbital) and crystal structures, and are being actively studied for understanding of their physical properties and industrial applications.
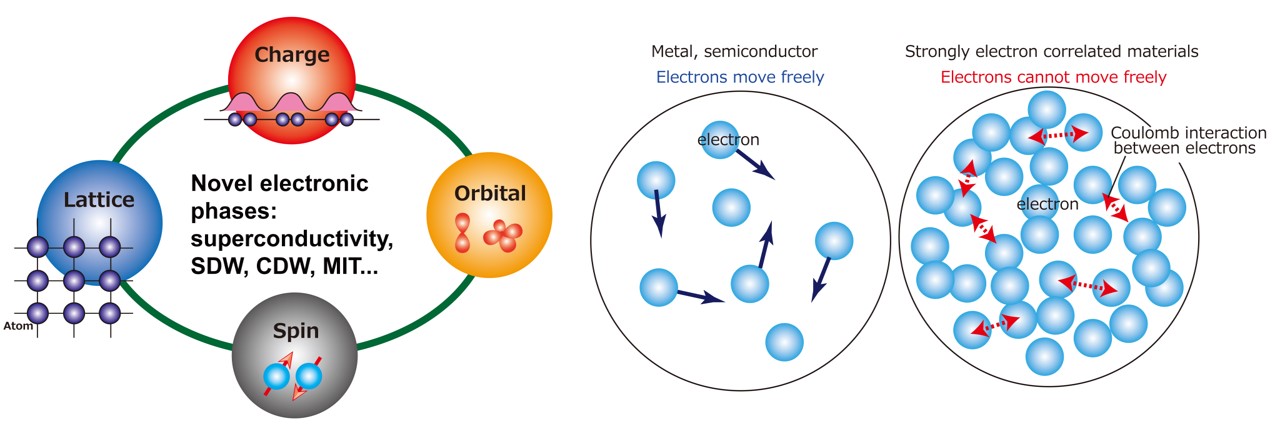
What is Superconductivity?
Superconductivity is a phenomenon in which electrical resistance becomes
zero at very low temperatures close to absolute zero (-273°C). This phenomenon
was discovered by Kamerlingh Onnes in 1908. It was experimentally proven
that superconductivity occurs at very low temperatures in various metals,
and the BCS theory was established in which electrons form pairs (Cooper
pairs) mediated by phonons. Furthermore, in 1986, it was reported that
metal oxides containing copper show superconductivity at about -200°C,
and it was found that the temperature at which superconductivity occurs
increases to nearly -100°C for metal oxides containing mercury (Hg). This
is a remarkable discovery that cannot be explained by the BCS theory and
overturns the conventional idea that superconductivity is exhibited only
at temperatures near absolute zero.
However, 35 years have passed since the discovery of high-Tc cuprate superconductors,
and we still do not know the origin of superconductivity, i.e., what is
the glue of Cooper pairs. In order to provide ideas for new materials with
higher superconducting transition temperatures, we need to study the electronic
structure in the superconducting or normal state in detail. Therefore,
angle-resolved photoemission spectroscopy (ARPES), which allows direct
observation of the electronic structure of materials, is a very powerful
tool to study the properties of strongly correlated electron systems.
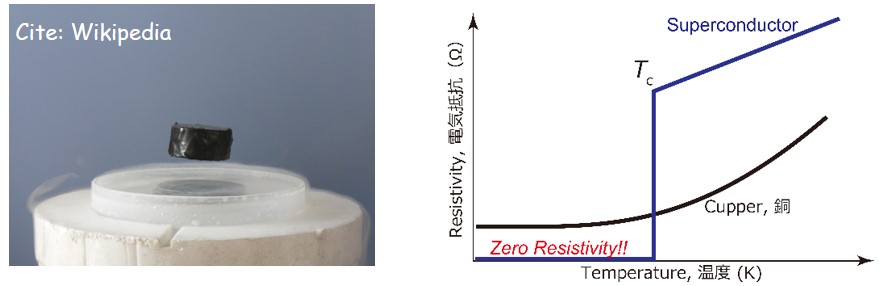
What is Topological Insulator?
What is topology anyway? This is a new concept that has emerged from research
over the last decade or so; many people first knew about it with the 2016
Nobel Prize in Physics. "Topology" is organized by the mathematical
concept. It is often used as an example of how a donut with holes and a
mug with a handle are isomorphic, meaning that they are "robust"
properties independent of details such as the size and shape of the holes.
Recently, real materials with such topological properties have been discovered
in the surface state. The bulk properties are insulating, but the surface
behaves like a metal, with spins moving in one direction (spin current)
and the flow unimpeded by impurities or lattice defects. These properties
have not been discovered in new materials, but have been demonstrated in
materials that have been known for a long time. Only in recent years has
the concept of topology been recognized and studied extensively. Basic
research for the development of spintronics materials and devices utilizing
spin currents has been actively developed.
Our research group is also studying the electronic structure of these
topological insulators.
Angle-Resolved Photoemission Spectroscopy (ARPES)
Angle-Resolved Photoemission Spectroscopy (ARPES), which we use in our research, is an experimental method established based on the "light quantum hypothesis" proposed by Einstein in 1905. When light is incident on a material, electrons inside the material are ejected as photoelectrons due to the external photoelectric effect. By examining the energy and position of these electrons in detail, the electronic state of the surface, edge, and bulk of the material can be directly investigated (left figure below). ARPES requires a change in sample angle in order to study momentum space. However, in BL-1 and BL-9A at HiSOR, by using the hemispherical electron analyzer with a deflector mode enables us to study tthe electronic structure in momentum space without changing the sample angle, so that even small samples can be observed. For details, please see the Beamline Introduction page.
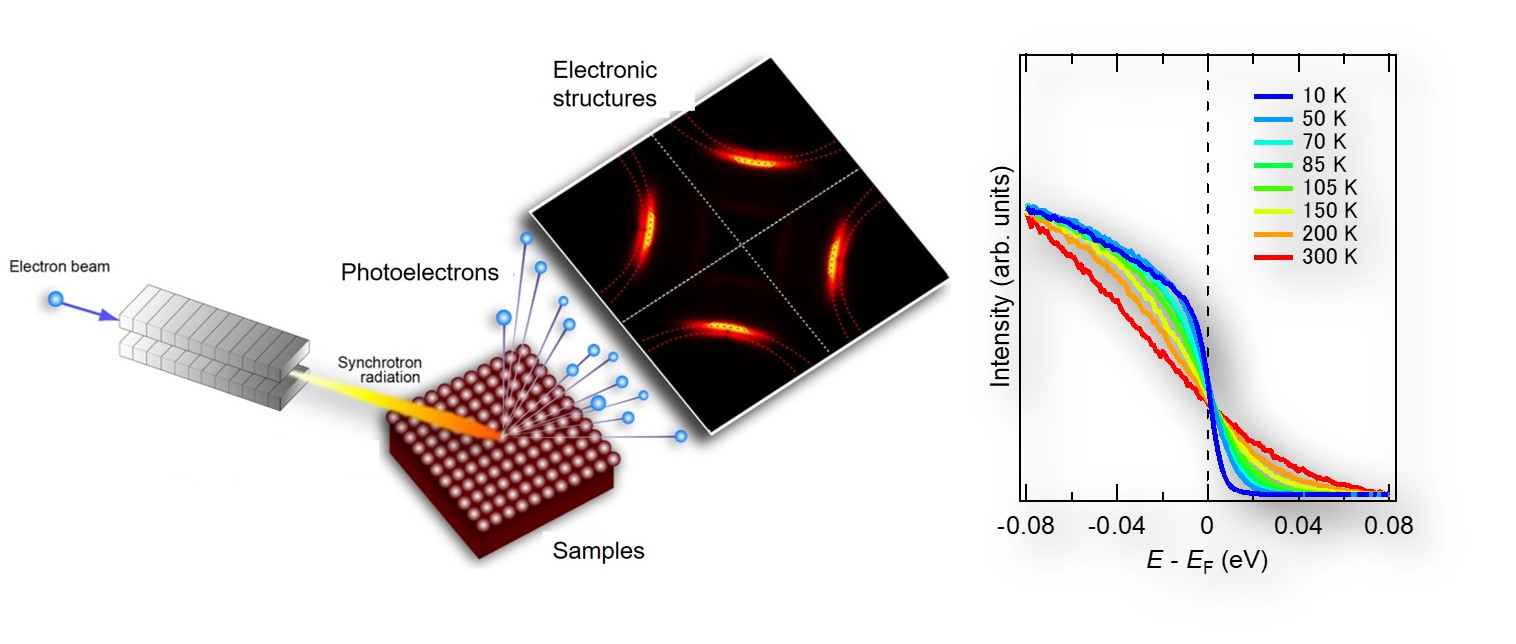
Spin-Resolved Photoemission Spectroscopy (SARPES)
This is an experimental method to observe the spin degree of freedom of
the electronic states in solids. Although it has been technically difficult
to separate the spin into spin-up and spin-down states, a spin-resolving
ARPES (SARPES) has recently been developed and tested by VLEED, which was
developed by the Spin Group at HiSOR [*]. This technique is very effective
in revealing spin-dependent electronic states.
In recent years, electronic devices such as the flash memory and DRAM
(Dynamic Rondom Access Memory) have been the focus of intense research
and development worldwide, and their performance has improved significantly.
These devices record data using an electron charge stored in a capacitor,
and there is a demand for the development of next-generation devices due
to read/write speed and volatility issues. Currently, a spintronics device,
MRAM (Magnetoresistive Random Access Memory), which controls not only charge
but also spin, the internal degree of freedom, is proposed as a new device
technology, and attention to electron spin is growing from an application
perspective as well. The spin of electrons is also attracting attention
from the viewpoint of applications.
[*] T. Okudaet al.,Rev. Sci. Instrum.82, 103302 (2011).
Introdution of Beamlines
The Hiroshima Synchrotron Radiation Center (HiSOR) at Hiroshima University is capable of performing experiments using high-brilliance, wavelength- and polarization-tunable synchrotron radiation from extreme ultraviolet (VUV) to soft X-rays. The Shimada/Ideta group mainly uses BL-1 (Shimada/Ideta group's beamline) and BL-9A. If you are interested in use, please contact the HiSOR office or the person in charge of each beamline.
BL-1
BL-1 is an experimental beamline that uses highly brilliant and polarization-tunable
synchrotron radiation with hν = 23 eV to 300 eV to study the electronic
structure of bulk solids and surfaces [**]. In addition to angle-resolved
photoemission spectroscopy (ARPES) using the deflector mode, this experimental
station can observe spatially resolved electronic states with a beam focused
down to 40-50 μm. For polarization dependence (the s and p polarizations), the endstation can be rotated by 90 degrees to allow strictly
distinguishable measurements of the orbital symmetry. For additional details,
please contact the person on duty (contact information).
[**] K. Shimadaet al., Nuclear Instruments and Methods in Physics Research A467, 504 (2001).
BL-9A
BL-9A can perform low-energy ARPES of bulk solids and thin films using synchrotron radiation in the ultraviolet region (wavelength: λ~30-200 nm) of hν = 6.5 eV to 40 eV. BL-9A is capable of generating high-brightness synchrotron radiation with high-energy resolution.From October 2022, a hemispherical analyzer (ASTRAIOS 190, SPECS, acquisition angle: ± 20°) and a 6-axis manipulator are installed at the BL-9A endstation (measurable temperature:T~ 10-300 K). Operando measurements are also possible. With these facilities, BL-9A is capable of observing three-dimensional Fermi surfaces in order to understand physical properties, as well as low energy excited states such as changes in the electronic structure of materials at low and high temperatures. For details, please contact the person in charge of the beamline (M. Arita)。
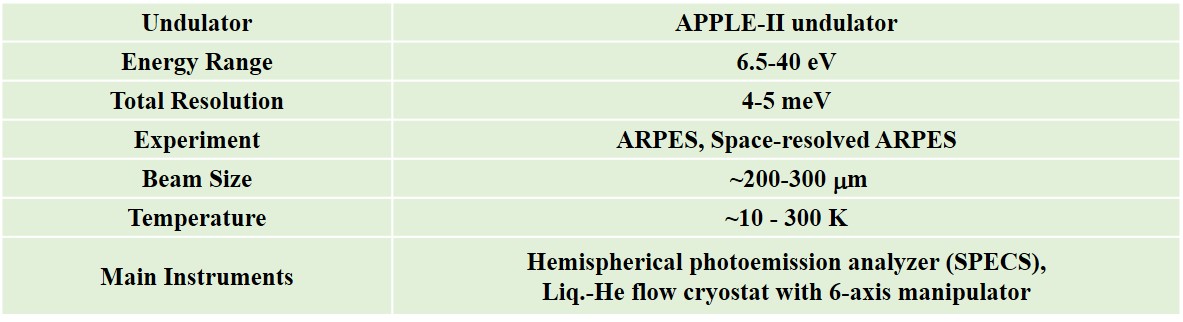